Functional materials, the kind that make up things like semiconductors, catalysts, photovoltaics, or batteries, don’t always arrive on schedule. In fact, they rarely do, according to a new paper in one of the inaugural issues of the journal Nature Synthesis. A funding agency can supply the dollars to conduct the research, and the research can get done, possibly creating a new material. But the big advancement, the kind that changes how we live and, occasionally, garners a Nobel Prize for the person given credit for it, often comes much later — decades later.
In their article, “Chemical Synthesis and Materials Discovery,” UC Santa Barbara professors emeritus Anthony K. Cheetham and Fred Wudl, together with Ram Seshadri, professor of chemistry, biochemistry, and materials and the director of the NSF-funded Materials Research Science and Engineering Center (MRSEC; aka the Materials Research Laboratory) at UCSB, examine the relationship between synthetic discoveries and the scientific breakthroughs that they have enabled. The paper tracks how and why the materials were initially synthesized and how — and when — their utility was eventually recognized.
The authors identify three pathways to materials breakthroughs. The less-common routes, they say, involve advances being made by using design principles informed by earlier work, or serendipitous breakthroughs, with the buckyball and Teflon being examples. The most common route to a breakthrough, however, appears not to involve inventing something new at all. Instead, an existing compound, often one that was synthesized out of simple curiosity with no idea of the eventual application, is repurposed for the application it becomes known for.
“The thesis of the article is basically to encourage discovery synthesis of materials without worrying too much about the application space,” say Cheetham and Seshadri. “We believe that a lot of scientists across the country will appreciate the case being made that you can’t overengineer research. Funding agencies would like to think that if they give you one dollar, then they will get one dollar — or ten dollars — of utility out of it. Actually, they may give you one dollar today that might pay back a million dollars twenty years from now, but it might be for something you never thought about or initially envisioned.
“Typically,” the authors write, “the synthetic discovery precedes the discovery of functionality by many decades; key examples include conducting polymers, topological insulators, and electrodes for lithium-ion batteries.”
The authors distinguish between the initial synthesis of a material — a “chemical discovery” that usually precedes a recognition of its useful functionality, with the latter considered a discovery in materials science. They provide several examples, such as formation of graphene from graphite, as well as the creation of quantum dots from semiconductors such as CdSe (cadmium selenide).
Polyacetylene, for example, was initially identified by Jacques-Martin Berthelot in 1866 and identified as a black powder called “cuprene,” and was first synthesized by a known polymer synthetic method in the 1950s. But it was only in the mid-1970s that that UCSB professor Alan Heeger, working with Alan MacDiarmid and Hideki Shirakawa (the three shared the 2000 Nobel Prize in chemistry) produced a revolutionary conductive thin film. That work led to the birth of the entire field encompassing applications of conjugated polymers, which include such areas as thermo-electrics and wearable electronics.
Hybrid 3D perovskites, another example of a delayed breakthrough application, were initially synthesize out of curiosity in Germany in 1978. Thirty-one years later, in 2009, the work was done that would lead to their application as visible-light sensitizers in photoelectrochemical cells, i.e. photovoltaics.
Lithium-ion batteries, too, are the multi-generational result of research on lithium cobalt oxide, which was first synthesized in the 1950s and found its way into service as the key compound in the battery that powers our remote electronic devices and our electric vehicles.
The authors note that some big advancements, such as the discovery of high-temperature copper oxide superconductors and the buckyball, both of which earned a Nobel Prize for their discoverers, have not directly led to large-scale commercial application — at least not yet. “This is not very surprising,” they write, “because important scientific discoveries do not necessarily lead directly to commercial applications.”
Finally, the authors explore developments in data mining and artificial intelligence (AI) for enabling materials discovery and discuss whether high-throughput computational approaches, coupled with high-throughput synthesis and AI methods, will eventually replace the role of chemical intuition and exploratory synthesis. They believe that it should not and conclude that funding agencies would be wise to recognize, from the historical record, that “curiosity-driven synthetic chemistry will be essential for the discovery of next-generation functional materials.”
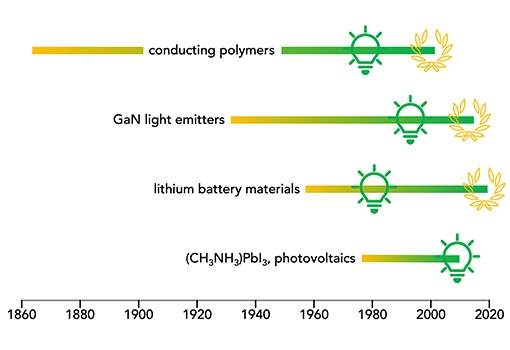
The start of the gradient line indicates the first chemical synthesis of the relevant material. The light bulb indicates the recognition of its importance. Laurels correspondence to the Nobel (top two are UCSB).