The 21st century presents the world with multiple sustainability challenges, especially on the energy front. Energy demand is growing rapidly, but fossil-fuel combustion drives climate change, creating a need to transform how we generate, supply, transmit, store, and use energy. Water is generally required to produce energy, and purifying water requires energy, increasing the urgency of finding energy-efficient ways to purify water and water-efficient ways to produce energy.
While incremental advances to existing technologies are helpful in these areas, such marginal improvements will not be enough to meet the future demands. Radical new technologies are needed, and, recognizing that advances at the frontiers of science will be required to produce them, in 2009 the U.S. Department of Energy (DOE) established the Energy Frontier Research Center (EFRC) program. Its goal: to establish the scientific foundation for a fundamentally new U.S. energy economy, one that will decisively enhance U.S. energy security and protect the global environment.
Since beginning the ambitious program, the DOE has established 36 integrated, multi-investigator EFRCs around the nation, involving partnerships among universities, national laboratories, nonprofit organizations, and for-profit firms. Each is conducting fundamental research focused on one or more “grand challenges” related to the shared energy future of the nation.
Now, scientists affiliated with UC Santa Barbara’s California NanoSystems Institute (CNSI) have joined that effort on four newly approved, renewable four-year EFRC projects.
ON THE WATER FRONT
The $10.75 million Center for Materials for Water and Energy Systems (M-WET) is a joint effort between UCSB ($3.9 million) and the project home, the University of Texas, Austin. Rachel Segalman, chair of the Department of Chemical Engineering, is the project associate director and PI on the UCSB team, which includes Mahdi Abu-Omar (chemistry), Christopher Bates (materials), Michael Doherty (chemical engineering), Glenn Fredrickson (materials, chemical engineering), Songi Han (chemistry, chemical engineering), Craig Hawker (materials, chemistry), Baron Peters (chemical engineering, chemistry), M. Scott Shell (chemical engineering), and Todd Squires (chemical engineering).
The M-WET team will take a materials approach, working at the atomic level to “design and perfect” revolutionary new forms that can be used as membranes for filtering chemically contaminated water for re-use. The researchers will seek to “master energy and information on the nanoscale to create new technologies having capabilities rivaling those of living things.“
Synthetic polymer membranes are widely used to purify water, mainly because they are more energy efficient than competing technologies. But current membranes were not designed for, and are not suitable for, applications involving water that is heavily contaminated with organic and inorganic components resulting from, for instance, oil and gas production.
In addition, water’s interactions with, and its structure near, complex heterogeneous surfaces (filtering membranes; see sidebar at right) remain poorly understood, and those gaps in knowledge limit the ability of scientists and engineers to develop materials for energy and water applications. M-WET is intended to fill those gaps, and the UCSB team will bring deep and broad expertise in polymer research to the project.
POWERING UP: DELIMITING LITHIUM ION BATTERIES
The Synthetic Control Across Length-scales for Advancing Rechargeables (SCALAR) is a collaboration with UCLA. The group, which includes UCSB PI Ram Seshadri (materials, chemistry) plus Brad Chmelka (chemical engineering), Anton Van Der Ven (materials), and Segalman, will take a materials approach to discovering the science that will enable more-powerful and energy-efficient batteries, which last longer and charge faster. Total funding for the project is $9.75 million, with UCSB researchers receiving just under $2 million for their part.
For more than two decades, lithium-ion batteries (LIBs) have had a profound impact on the development of a vast range of electronics that are important in all aspects of daily life. LIB market share is already enormous and will only grow with the electrification of transportation. In fact, electrified vehicles alone are expected to generate a nearly twenty-fold increase in LIB demand by 2025, and the integration of grid-level energy storage for renewables will further extend market dominance.
The materials used in LIBs work surprisingly well, but new materials are needed for better performance that will enable technological advances, whether it’s making smaller, longer-lasting devices or electrifying larger modes of transportation, such as airplanes. Moreover, the ability to move to truly renewable energy sources — e.g. wind, solar, and hydro — requires improved battery storage capacity to handle the time-dependent availability of the energy generated by those sources.
Recognizing the limitations of LIB technology and the challenges they present, the SCALAR team has received funding for an integrated four-year program to create the enabling science and technology for next-generation electrochemical energy storage.
“Energy storage in batteries has become an increasing concern that can enable numerous future technologies, including those associated with renewable and clean-energy technologies,” says Seshadri, who is also director of the NSF-funded Materials Research Science and Engineering Center (aka, the Materials Research Laboratory) at UCSB. “UCSB has great depth of expertise in this area, and the EFRC is an opportunity to bring together a number of experts to address these important challenges. The UCSB team is particularly happy to combine expertise in soft (e.g. polymer) and hard (e.g. oxide) materials to improve the performance of lithium ion batteries.”
TOWARD CONTROLLING QUANTUM COHERENCE
Physicist Ania Bleszynski Jayich is the sole UCSB researcher on another EFRC project, titled Novel Pathways to Quantum Coherence in Materials and based at the Lawrence Berkeley National Laboratory. She explains that a key goal of her part in the $11.75 million project, $540,000 of which is directed toward her research, is to “dramatically expand our understanding of quantum coherence in solids by building on fundamental materials discoveries.”
Quantum systems, such as atoms, electrons, and photons, are unique in that they can exist in what is called a superposition state. That is, they can be in two places at once. Quantum coherence refers to this fleeting phenomenon, which has no limit to how briefly it may last before dissipating into a classical state in a process called quantum decoherence. Quantum computing, quantum communication, and other quantum applications all take advantage of, and are made possible by, the superposition state.
In recent years, considerable progress has been made in creating systems that exhibit quantum coherence for measurable periods of time, on the order of microseconds and milliseconds. Jayich explains: “That has led to an explosion of research in the field of quantum technologies, where researchers utilize these quirky, non-intuitive phenomena of quantum mechanics to realize functionalities that are not enabled by classical physics.”
For quantum technologies to be functional, quantum coherence has to exist for at least a few nanoseconds, long enough to perform a logic operation or store information in memory. “You need to do those things faster than the quantum bit loses its ‘quantumness,’” Jayich notes. “For instance, if I need to send you some encrypted information, then in the time frame lasting from the moment I send the information to you until you receive it, everything has to be quantum coherent. That's critical to enabling these technologies.”
The “novel pathways” part of the project title refers to developing new methods for maintaining quantum coherence, addressing the fact that coherence is highly susceptible to environmental factors. “If you do it just right, you can understand what the decohering interactions with the environment are and fine-tune the system to stabilize coherence,” she says.
“The materials approach means looking for materials that preserve coherence, looking at the defects in them and trying to improve them, kind of cleaning up the materials and characterizing them."
GREAT MINDS: THE NEUROMORPHIC COMPUTER
Back in the 1970s, “Moore’s Law” posited a doubling of the number of transistors on an integrated circuit every two years, and a corresponding increase in processing speed. And while it has held true for several decades, experts agree that in the next 15 to 25 years, that cycle will end. With more data available all the time, a new computing paradigm is needed.
Associate Professor Jon Schuller is the one UCSB researcher on an EFRC project — Quantum Materials for Energy-Efficient Neuromorphic Computing (Q-MEEN-C) — directed at identifying, developing, and characterizing quantum materials to enable energy-efficient “neuromorphic” computing. A neuromorphic computing architecture would make it possible to solve problems in a way that replicates how the human brain functions. Total funding for the four-year project is $9.75 million with $200,000 directed at Schuller’s work.
Based at UC San Diego, the Q-MEEN-C project is meant to establish fundamental research to drive the next information revolution. The grand challenge is to develop a highly energy-efficient and fault-tolerant computational machine “that works like the brain.”
Conventional materials, devices, and architecture are not up to that task, because they are comparatively energy-inefficient (the brain uses very little energy), are strongly affected by defects (the brain is very good at accommodating and sorting through defects), and have reached fundamental limits in terms of size and processing speed (the end of Moore’s Law). Q-MEEN-C researchers will explore and seek to understand and control the novel physical mechanisms of quantum materials that can enable functionalities necessary to develop a neuromorphic machine.
“By mimicking aspects of the brain and using them in your computer architecture, you can unlock the ability to process energy efficiently, and new materials are the key to that,” Schuller says.
As an expert in conducting optical spectroscopy of quantum materials and demonstrating their use in reconfigurable devices, Schuller will be a valuable contributor in terms of identifying and characterizing potentially useful materials. He collaborated previously on an internal UC multi-campus grant, working with four UC San Diego colleagues who are also part of Q-MEEN-C.
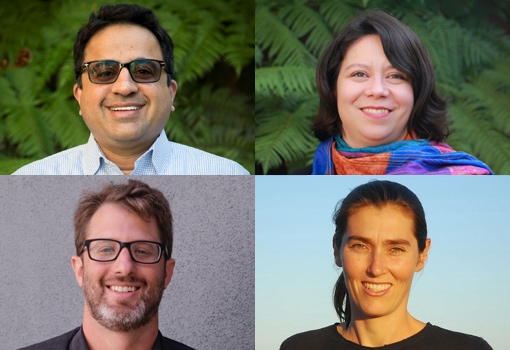
(Clockwise from top-left) Ram Seshadri , Rachel Segalman, Ania Bleszynski Jayich, and Jon Schuller