UC Santa Barbara professors of electrical and computer engineering Galan Moody and John Bowers, as well as collaborators from the California Institute of Technology, the Massachusetts Institute of Technology, the University of Virginia, and Nexus Photonics have received a nearly $10-million grant from the U.S. Department of Defense (DoD) through the Defense Advanced Research Projects Agency (DARPA) to develop next-generation optical detectors.
The project, part of the DARPA Intensity-Squeezed Photonic Integration for Revolution Detectors (INSPIRED) program, will push the limits of detection capabilities through transformative advances in sensitivity, size, weight, and power consumption (SwaP) — key factors in applications such as medical imaging, remote sensing, navigation, microscopy, and communications. The team will achieve this by utilizing compact integrated photonic chips that generate squeezed light — a special kind of light that can reduce the noise on optical detectors. Decreasing such noise would increase the likelihood of advanced optical technologies being deployed in what the DoD refers to as “SWaP-constrained” systems; i.e. devices that are powerful, precise, and far smaller than their forerunners, and that can detect even the faintest optical signals.
“The nonlinear effects that generate squeezed light are more efficient in chip-scale devices than in bulk devices, thanks to stronger field confinement in the former,” Bowers notes. “Furthermore, the coupling losses are lower, so that the squeezed state is preserved. The inherent stability of solid-state photonic circuits and the flexibility that is possible in designing components may also provide significant performance advantages in manipulating quantum states of light.”
Low-noise detectors are vital in sophisticated optical-science experiments and related technologies, since noise can degrade the measurement accuracy and ability to detect weak signals. One primary way to increase the signal-to-noise ratio (SNR) is to increase the signal power by amplifying the signal or using larger collection optics, but those capabilities are not available in many contexts, because they can be power-hungry and require large, heavy components. Conversely, decreasing noise in optical detection makes it possible to shrink the systems and deploy them in SWaP-constrained platforms. Yet, while designers of optical systems make great efforts to eliminate noise, conventional methods of optical measurement are ultimately constrained by quantum mechanics. This quantum-limited sensitivity is due to the intrinsic, random intensity fluctuations of laser light used for measurements or communication signals, typically referred to as shot noise.
This quantum limit in optical detection noise is often regarded as a fundamental constraint, but in the past two decades, sophisticated experiments have utilized squeezed light to reduce the noise to below this limit. The most notable example is LIGO — the laser-interferometer gravitational-wave observatory — which is a few-kilometer-sized experiment designed to study the universe. LIGO uses interference between two powerful laser beams to measure tiny stretching of spacetime due to cosmic gravitational waves that originated from high-energy events more than a billion light years away.
“Advanced LIGO is now using squeezed light that makes it possible to peer deeper into the universe,” says Moody, the lead investigator on the project. By injecting squeezed light into the interferometer, LIGO researchers are able to reduce the noise on their detectors below the quantum limit. “We hope to replicate some of their successes in developing really sensitive optical detectors that incorporate squeezed light, but with compact, chip-scale components integrated into a portable module that you can hold in your hand.” UCSB has teamed up with members of the MIT-LIGO team, who will assist in the device designs, measurements, and analysis to make sure the detectors operate with optimal performance.
The team’s designs will utilize integrated photonics, which are small semiconductor chips that can generate, route, manipulate, and detect light — the specialty of the Moody and Bowers groups as well as collaborators at Nexus Photonics and the Caltech.
Sophisticated integrated-photonics technologies based on silicon nitride and III-V semiconductor waveguiding materials will be utilized to generate squeezed light and route it around the chip to high-efficiency optical detectors to enhance their sensitivity. And all of this will happen in a compact module about the size of a credit card, and it will include integrated microelectronics developed by collaborators at the University of Virginia.
These advances could have a powerful impact in many different fields, from biomedical imaging to space exploration, navigation, and quantum computing. The project will be funded in two phases, with the first focusing on developing the integrated photonic and microelectronic technologies, and the second focusing on full system integration.
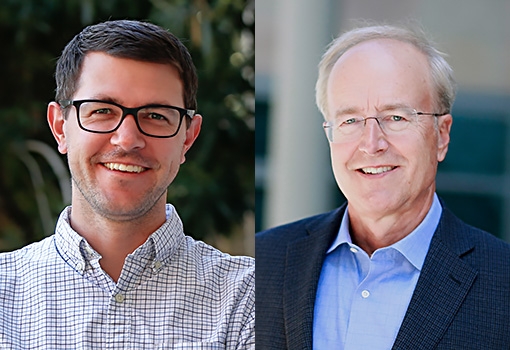
Galan Moody (left) and John Bowers