The dynamics of water near solid surfaces play a critical role in numerous technologies, including water filtration and purification, chromatography, and catalysis. One well-known way to influence those dynamics, which in turn, affects how water “wets” a surface, is to modify the surface hydrophobicity, or the extent to which the surface appears “oily” and repels water. Such modifications can be achieved by altering the average coverage, or surface density, of hydrophobic chemical groups on the interface.
Now, in a paper published in the Proceedings of the National Academy of Sciences, lead author Jacob Monroe, a fifth-year PhD student in the lab of UCSB chemical engineering professor M. Scott Shell, describes a new perspective on the factors that control water dynamics at interfaces. The findings could have important ramifications for membranes, especially those used in water filtration.
“What we’re seeing is that just changing the patterning alone — the distribution of those hydrophobic and hydrophilic groups, without changing the average surface densities — produces fairly large effects at an interface,” Monroe said. “That’s valuable to know if I want water to flow through a membrane optimally.”
Monroe and Shell found that if they arranged all the hydrophobic groups together and made the surface very patchy, the water moved faster, but if they spread them all apart, the water slowed down. “If the membrane were for water filtration, you might want the water to move quickly across it,” Monroe notes, “but you might also want to sit at the surface to repel par-ticles that stick to it and foul the membrane.”
Monroe's finding about patterning holds immediate relevance for interpreting experiments, because it means that assessing the surface density of hydrophobic groups alone is not enough to characterize the material.
Monroe and Shell discovered the distribution effect by combining simulations of molecular dynamics with a genetic algorithm optimization, which is simply an algorithm that emulates natural evolution — here used to identify surface patterns that either increase or decrease surface-water mobility.
“It’s kind of like a breeding program,” Monroe explains. “If you had a pool of dogs and wanted a certain kind of dog, say one that’s bigger or has a shorter tail, you would breed the dogs that have those characteristics. We do the same thing on a computer, but our goal is to design a surface having specific characteristics that allow it to perform how we want it to. You need the fitness metric, and then you can tune the genetic algorithm to optimize specific performance characteristics, for instance, to have water move quickly across a membrane or to adsorb on a surface.
“We run molecular dynamics simulations to assess those properties,” he adds. “We assign a level of fitness to each individual, and then hybridize the most fit individuals spatially and drive the systems toward the desired properties.
“This work is exciting because it shows for the first time that nanoscale patterning on surfaces is an effective means of engineering materials that give rise to unique water dynamics,” Shell says. “It has long been thought that biological molecules, like proteins, use surface chemical patterning to influence water dynamics toward functional ends, such as accelerating binding events that underlie many biomolecular processes. We have now used a computational optimization algorithm to 'learn' what these patterns should look like in synthetic materials having target performance characteristics. The results suggest a new way to design surfaces to precisely control water dynamics near them, which becomes widely important to chemical separations and catalysis tasks.”
The research will also be useful in a new Energy Frontier Research Center (EFRC) project (see article at left). In that effort, the researchers will be taking a materials approach to “design and perfect” revolutionary new materials that can be used as membranes for filtering chemically contaminated water for re-use.
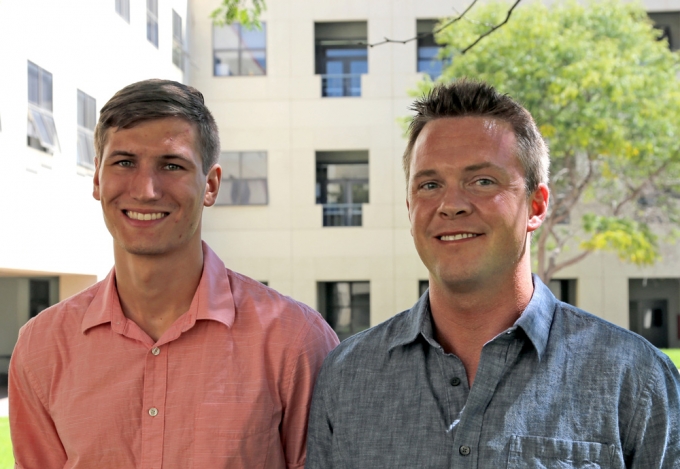
Jacob Monroe (left) and M. Scott Shell